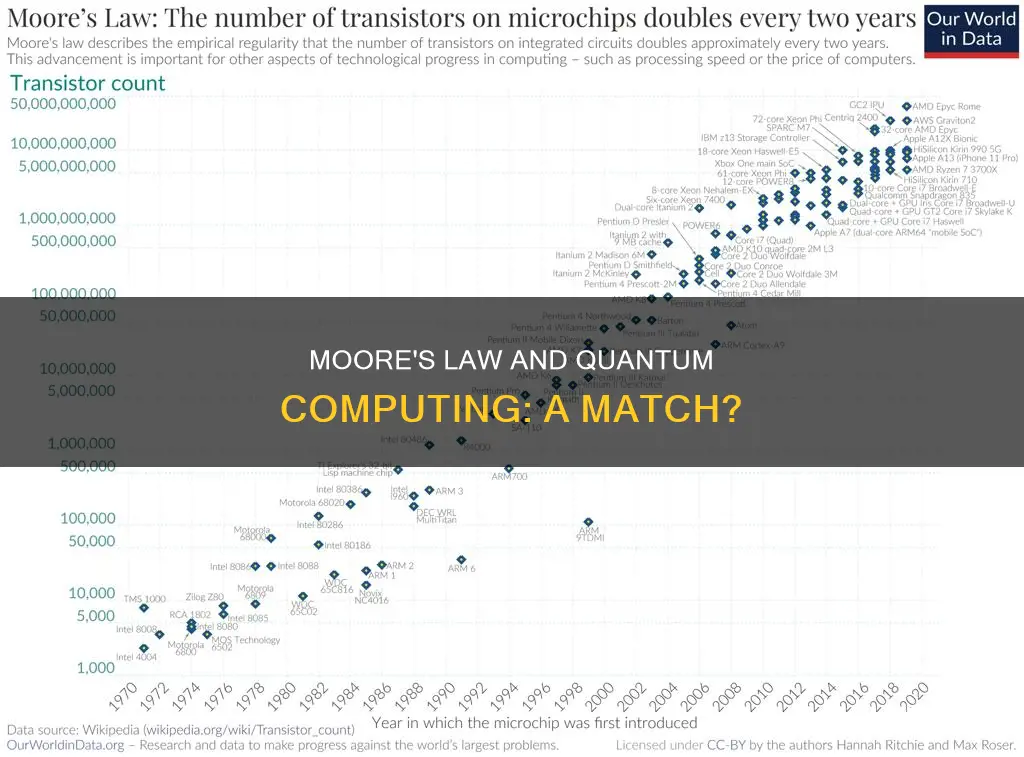
Moore's Law states that the number of transistors in a dense integrated circuit doubles about every two years. This observation, made by Intel co-founder Gordon Moore in 1965, has ruled the chip-based computing industry for decades. But does it apply to quantum computing?
According to Robert Sutor, IBM's chief quantum exponent, Moore's Law does not apply to quantum processors. This is because quantum bits, or qubits, have a weird property called entanglement. By adding one more qubit to a system, you double the amount of information that your quantum system can compute. This is exponential growth, similar to Moore's Law, but it is not the same.
While Moore's Law is based on the physical limitations of transistors, qubits are not limited by physical space in the same way. They are also subject to the strange laws of quantum mechanics, which do not apply to classical computing bits.
However, this does not mean that there is no correlation between Moore's Law and the development of quantum computing. According to Hartmut Neven, director of Google's Quantum AI Lab, quantum computing power is improving at a doubly exponential growth rate compared to conventional computing. This means that quantum computing power is growing by powers of powers of two.
While Neven's prediction is enticing, it is based on limited data and does not consider the technical problems that will arise as processors become more complex. Only time will tell if a similar law to Moore's will apply to quantum computing.
Characteristics | Values |
---|---|
Definition of Moore's Law | The number of transistors in a dense integrated circuit doubles about every two years |
Applicability of Moore's Law to Quantum Computing | Moore's Law does not apply to Quantum Computing |
Reason for Inapplicability | Moore's Law is not a law of fundamental physics but an observation of a stablished industry. There is no stablished industry producing quantum computers |
Applicability of a Modified Moore's Law | A modified Moore's Law may apply if the quantum computing industry finds ways to iteratively improve the components' manufacturing process to exponentially increase their count |
Quantum Computing Performance | Quantum computers are already 100 trillion times faster than conventional supercomputers for certain specialized tasks |
Quantum Computing Benchmarking | The concept of benchmarking in quantum computing is still in its infancy |
Quantum Computing and Exponential Growth | Quantum computers appear as an exponential function on a logarithmic scale. Quantum computers are inherently and exponentially better than classical computers |
What You'll Learn
Quantum computers are designed differently to traditional computers
Quantum computers are designed differently from traditional computers in several ways. Firstly, they harness the unique qualities of quantum mechanics to solve problems that are beyond the capabilities of classical computers. This includes leveraging the principles of superposition, entanglement, decoherence, and interference to compute probabilistically and quantum mechanically.
Secondly, quantum computers use qubits instead of classical bits to store and process data. Qubits can exist in a superposition of states, meaning they can represent both 0 and 1 simultaneously. This allows quantum computers to encode and process exponentially more data compared to classical computers.
Thirdly, quantum computers process data with quantum logic in parallel instances, relying on interference. In contrast, classical computers process data logically and sequentially. Quantum computers can process enormous datasets simultaneously with different operations, improving efficiency by several orders of magnitude for certain complex problems.
Additionally, quantum computers are probabilistic, finding the most likely solution to a problem, while classical computers are deterministic, requiring step-by-step computations to determine a specific outcome. This means that quantum computers often provide a range of possible answers, whereas classical computers typically provide a singular answer.
Lastly, quantum computers require specialised hardware and extreme cooling systems to minimise noise and prevent decoherence. Qubits are extremely sensitive to environmental factors and must be carefully isolated to maintain their delicate quantum states.
Understanding Labor Laws: Salaried Employees' Rights Explained
You may want to see also
Moore's Law is not a law of physics
In 1965, Intel co-founder and CEO Gordon Moore observed that the number of transistors on an integrated circuit seemed to double at regular intervals. Initially, Moore's statements were economic, noting that the cost per component is nearly inversely proportional to the number of components.
Moore's Law is a projection of a historical trend. It states that the number of transistors in an integrated circuit (IC) doubles about every two years. However, it is not a scientific law but rather an observation and prediction of future trends based on past behaviour.
Moore's Law has been a driving force in the semiconductor industry, guiding long-term planning and setting targets for research and development. It has influenced technological and social change, productivity, and economic growth.
While Moore's Law has held true for several decades, it is not a fundamental physical law. It is based on human behaviour and creativity, as well as advancements in technology.
Moore himself acknowledged in a 2005 interview that the law would not hold indefinitely and that there were fundamental limitations due to the atomic nature of materials.
Marijuana and Three Strikes: Law Application?
You may want to see also
Quantum computers are in their infancy
The fundamental unit of computation in traditional electronics is the bit, which can be either 0 or 1. Quantum computers, on the other hand, use qubits, which can be both 0 and 1 at the same time, a property known as quantum superposition.
While Moore's Law states that the number of transistors on an integrated circuit doubles every two years, it does not apply to quantum computers. This is because quantum computers are designed very differently, based on the laws of quantum physics.
However, this does not mean that quantum computers are not rapidly improving. Hartmut Neven, the director of Google's Quantum AI Labs, has proposed "Neven's Law", which states that quantum computing power is improving at a doubly exponential growth rate compared to conventional computing. This means that quantum computing power is growing by powers of powers of two.
While Neven's Law is based on a small number of prototypes and a short timeframe, it is widely believed that progress in quantum computing is being made at an exciting pace. However, it is important to note that as quantum processors become more complex and powerful, technical problems, such as electrical noise, could become more significant and affect the accuracy of the processors.
Therefore, while quantum computers are still in their infancy, their potential to revolutionise computing power is undeniable, and further advancements in this field are eagerly anticipated.
HIPAA Laws: Do They Apply to Spouses?
You may want to see also
Quantum computers are exponentially faster than traditional computers
Quantum computers are built on the laws of quantum physics, and they are designed very differently from traditional computers. Traditional computers store data as electrical signals that can have one of two states (1 or 0), but quantum computers can use physical systems like electrons and photons to store data. This allows quantum computers to encode information in multiple states, enabling them to perform calculations exponentially faster than traditional computers.
The exponential growth in the power of traditional computers is described by Moore's Law, which states that the number of transistors that can be integrated into a silicon microchip doubles roughly every two years. However, Moore's Law does not apply to quantum computers due to their unique design.
The director of Google's Quantum AI Labs, Hartmut Neven, has proposed "Neven's Law" to describe the growth of quantum computing power. Neven's Law states that quantum computing power is improving at a doubly exponential rate compared to conventional computing. This means that quantum computing power is growing by powers of powers of two: 2^2 (4), 2^4 (16), 2^8 (256), and so on.
The reason for this doubly exponential growth is the quantum effect called entanglement, which allows different computational tasks to be performed simultaneously, resulting in exponential speedups. Each additional qubit added to a quantum system doubles the amount of information it can compute. This is similar to Moore's Law's exponential growth but with a crucial difference: in a quantum system, there is no corresponding physical space for these qubits to occupy.
While Neven's Law shows promise, it is important to exercise caution as it is based on a small number of prototypes and a relatively short timeframe. Additionally, as quantum processors become more complex, technical issues such as electrical noise can become more significant and impact computational accuracy.
Artisan Labor Laws: Do NGOs Have Exemptions?
You may want to see also
Quantum computers are not widely commercialised
Secondly, quantum computers are designed very differently from classical computers, following the laws of quantum physics. This means that Moore's Law, which describes the exponential growth of traditional computing power, does not apply to quantum computers. While a quantum version of Moore's Law has been proposed, known as Neven's Law, it is based on limited data and may not accurately predict the future of quantum computing.
Thirdly, there are technical challenges unique to quantum computers that could become more significant as they become more complex. For example, electrical noise, which is a minor issue in classical computers, could lead to computational errors in quantum systems as the processor complexity increases. Implementing error correction protocols would require adding redundant backup hardware, increasing the complexity without a proportional increase in power.
Finally, it is worth noting that even if quantum computers do become widely commercialised, they may not replace classical computers entirely. Classical computers are better suited for tasks that are parallel or sequential, such as graphics processing. Quantum computers, on the other hand, excel at searching all possible inputs or optimising within constraints, making them ideal for code-breaking, AI optimisation, and quantum chemistry applications.
In conclusion, while quantum computers hold immense potential, they are not yet widely commercialised due to the challenges of benchmarking, the unique technical limitations, and the specialised nature of quantum computing applications.
Kepler's Laws: Beyond the Six Planets
You may want to see also
Frequently asked questions
No, Moore's Law does not apply to quantum computing. Moore's Law states that the number of transistors in a dense integrated circuit doubles about every two years. However, quantum computers do not have transistors, so this law does not apply to them.
Moore's Law is specific to the number of transistors in a dense integrated circuit, which is not a component of quantum computers. While it may be tempting to draw parallels between the exponential growth described by Moore's Law and the potential growth of quantum computing, it is important to remember that Moore's Law is not a fundamental law of physics but rather an observation of a specific industry at a specific time.
Moore's Law is based on the number of transistors in a dense integrated circuit, while quantum computing power is based on the number of qubits, which are the quantum counterpart to classical computer bits. Qubits have unique properties, such as the ability to be both 0 and 1 at the same time (superposition) and to entangle with other qubits, enabling exponential growth in computational power.
Yes, there have been attempts to create similar laws for quantum computing, such as Rose's Law and Neven's Law. Rose's Law suggests that the number of qubits in quantum computers will double every two years, while Neven's Law proposes that quantum computing power is improving at a doubly exponential growth rate compared to conventional computing.
One challenge is that quantum computing is still in its early stages and does not yet have a established industry producing quantum computers on a large scale. Additionally, quantum computers face technical challenges, such as the presence of electrical noise, which can lead to computational errors, and the need for error correction protocols, which can increase the complexity of the system without adding significant power.