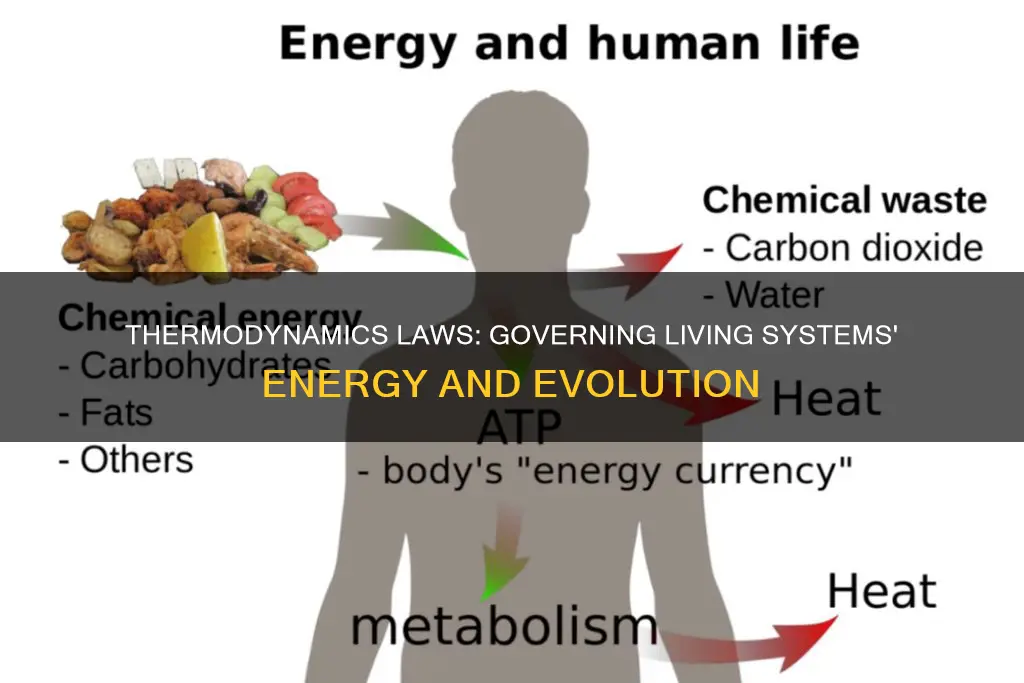
The laws of thermodynamics are fundamental to our understanding of the universe and the biological systems within it. The first law of thermodynamics, also known as the law of conservation of energy, states that energy cannot be created or destroyed, only transformed. This law applies to all biological organisms, which require a constant input of energy to survive. The second law of thermodynamics states that when energy is transferred, there will always be a loss of energy, resulting in an increase in entropy or disorder. Living systems, therefore, are in a continuous battle against the constant increase in entropy. This battle is fought by the input of energy, which allows living organisms to maintain their highly ordered, low-entropy state.
Characteristics | Values |
---|---|
The First Law of Thermodynamics | States that the total amount of energy in a closed system is constant; energy can be transferred or transformed but not created or destroyed |
The Second Law of Thermodynamics | States that every energy transfer involves some loss of energy in an unusable form, resulting in a more disordered system |
Living Organisms | Are open systems that require a constant input of energy to maintain their highly ordered, low-entropy state |
Entropy | A measure of randomness or disorder in a system; the higher the entropy, the higher the disorder and the lower the energy |
What You'll Learn
The First Law of Thermodynamics and living systems
The First Law of Thermodynamics, also known as the law of conservation of energy, states that energy can neither be created nor destroyed. It may change from one form to another, but the total amount of energy in the universe remains constant. This law applies to living systems, which are never in equilibrium and require a constant input of energy to survive.
Living systems, such as biological organisms, are thermodynamic systems of an active type, in which energy transformations occur. They are not closed systems, as they have inputs and outputs of matter and energy. However, the First Law of Thermodynamics still applies to them, as it governs the chemical processes (metabolism) that occur within them.
In biological systems, energy transformations occur through the conversion of the energy of the sun and food into other types of energy. For example, during photosynthesis, plants absorb light energy from the sun and convert it into chemical energy stored within organic molecules. This chemical energy is then released through cellular respiration, allowing plant and animal organisms to access and use it to perform essential cell functions such as DNA replication, cell movement, and apoptosis.
The First Law of Thermodynamics also applies to the processes within living cells. Chemical energy stored within organic molecules such as sugars and fats is transformed through a series of cellular chemical reactions into energy within molecules of ATP (adenosine triphosphate). This energy is easily accessible and allows cells to perform various types of work, including building complex molecules, transporting materials, and reproducing.
The First Law of Thermodynamics, therefore, plays a crucial role in understanding the energy dynamics within living systems, from the cellular level to entire organisms. It highlights the importance of energy conservation and transformation in maintaining the highly ordered state of living organisms.
Deer Hunting Laws on Private Kentucky Property Explained
You may want to see also
The Second Law of Thermodynamics and living systems
The Second Law of Thermodynamics states that when energy is transferred, there will be less energy available at the end of the transfer process than at the beginning. This is due to the presence of entropy, which is a measure of disorder in a closed system. As energy is transferred, entropy increases.
Living systems are highly ordered and have low entropy. To maintain this order, living systems require a constant input of energy. However, the processes performed to maintain this order result in an increase in entropy in the system's surroundings. This is because some energy is lost to the surroundings or transformed. For example, in the process of photosynthesis, not all of the light energy is absorbed by the plant—some is reflected, and some is lost as heat.
The second law of thermodynamics, therefore, applies to living systems in that they must constantly battle against the increase of entropy. Living organisms maintain their non-equilibrium states by coupling their activities to the non-equilibrium condition of the surrounding physical environment. They capture energy from the continuous flow of energy from the sun to the earth and use it to create temporary organisation and structure.
The ability of living organisms to grow and increase in complexity does not contradict the second law. In fact, under some definitions, an increase in entropy can result in an increase in complexity. Furthermore, for a finite system interacting with finite reservoirs, an increase in entropy is equivalent to an increase in correlations between the system and the reservoirs.
The stability of the non-equilibrium thermodynamic state of biological systems is ensured by the continuous alternation of phases of energy consumption and release through controlled reactions of synthesis and cleavage of adenosine triphosphate (ATP). This principle was formulated by Boris Dobroborsky as the second law of thermodynamics of biological systems:
> The stability of the non-equilibrium thermodynamic state of biological systems is ensured by the continuous alternation of phases of energy consumption and release through controlled reactions of synthesis and cleavage of ATP.
Stark Law and Pharmacies: Understanding Legal Compliance
You may want to see also
Entropy and living systems
The laws of thermodynamics are important unifying principles of biology, governing the chemical processes (metabolism) in all biological organisms. The Second Law of Thermodynamics states that when energy is transferred, there will be less energy available at the end of the transfer process than at the beginning. This is due to entropy, which is the measure of disorder in a closed system. Entropy increases as energy is transferred.
Living systems require a constant input of energy to maintain their highly ordered state. For example, cells are highly ordered and have low entropy. However, the processes performed to maintain this order result in an increase in entropy in the cell's/organism's surroundings. The transfer of energy causes entropy in the universe to increase.
The concept of entropy is particularly relevant when considering the origin and evolution of life. In 1944, Erwin Schrödinger published the book "What is Life?", in which he stated that life decreases or keeps constant its entropy by feeding on negative entropy, or negentropy. Schrödinger's idea was that what differentiates life from other forms of matter is that life creates ordered biological structures by increasing local entropy.
Recent work has focused on the concept of Gibbs free energy, rather than entropy, in relation to the thermodynamic interpretation of evolution. This is because biological processes on Earth occur at a constant temperature and pressure, and the Gibbs free energy is a useful way to express the Second Law of Thermodynamics in these conditions. The minimization of Gibbs free energy is a form of the principle of minimum energy, which follows from the entropy maximization principle for closed systems.
Living systems maintain or increase local order by working against the Second Law of Thermodynamics. They do this by consuming free energy, thereby increasing the net entropy of their environment. Entropy production estimators have been developed to quantify the rate of entropy production in living systems, providing insights into the efficiency of cellular processes.
Entropy and the laws of thermodynamics are fundamental to our understanding of living systems. Living organisms maintain their highly ordered state by consuming energy and increasing the entropy of their surroundings. The study of entropy in living systems has implications for our understanding of the origin and evolution of life, as well as for various fields such as psychology and information theory.
Kirchhoff's Loop Law: Wattage and Voltage Explained
You may want to see also
Energy transfer in living systems
The laws of thermodynamics govern the chemical processes, or metabolism, in all biological organisms. The First Law of Thermodynamics, also known as the law of conservation of energy, states that energy can neither be created nor destroyed. In other words, energy can be transferred or transformed but the total amount of energy in the universe remains constant. This is true of all closed systems, where energy cannot be transferred to the surroundings.
Biological organisms, however, are open systems, where energy can be exchanged between the system and its surroundings. They are able to consume energy-storing molecules and release energy to their environment by doing work. For example, plants convert light energy from the sun into chemical energy, which is stored in the form of glucose. This energy can then be released through cellular respiration, allowing plant and animal organisms to access the energy stored in carbohydrates, lipids, and other macromolecules.
The Second Law of Thermodynamics states that when energy is transferred, there will be less energy available at the end of the transfer process than at the beginning. This is due to an increase in entropy, or disorder, within the closed system. All energy transfers result in some loss of usable energy, often in the form of heat energy. This means that living systems require a constant input of energy to maintain their highly ordered, low-entropy state.
Living systems are therefore in a continuous battle against the constant increase in universal entropy. They must take in energy-storing molecules and transform them through chemical reactions, which requires an input of energy. The formation of macromolecules from smaller molecules, for example, requires an input of energy to force disordered atoms into ordered, non-random positions. This energy is stored in the bonds between molecules or macromolecules and can be released to do work.
The transfer and transformation of energy are fundamental to all biological organisms and are governed by the laws of thermodynamics.
International Criminal Law: Global Reach and Application
You may want to see also
Energy efficiency in living systems
Energy efficiency is a cornerstone of many sustainable energy strategies. It is the process of reducing the amount of energy required to provide products and services. It is called the "first fuel" in clean energy transitions, as it provides some of the quickest and most cost-effective carbon mitigation options while lowering energy bills and strengthening energy security.
Energy efficiency is the single largest measure to avoid energy demand in the Net Zero Emissions by 2050 Scenario. Most efficiency measures result in cost savings to consumers, lowering energy bills and helping cushion the effects of unexpected price spikes.
Energy efficiency is central to energy policy in Germany. In Spain, four out of every five buildings use more energy than they should. The Unión de Créditos Immobiliarios (UCI) is increasing loans to homeowners and building management groups for energy-efficiency initiatives. In May 2016, Poland adopted a new Act on Energy Efficiency.
In the United States, the 2022 Inflation Reduction Act includes major investment support for energy efficiency. For example, the High-Efficiency Electric Home Rebate Act provides up to USD 14,000 per household for upgrades to heating, ventilation, air conditioning systems, and more.
China strengthened its industrial energy efficiency policies in 2022 with new plans to improve the energy intensity of the sector by 13.5% by 2025 compared with 2020 levels.
In 2016, the German government released a green paper on energy efficiency for public consultation. It outlines the potential challenges and actions needed to reduce energy consumption in Germany over the coming decades.
In July 2009, the Council of Australian Governments agreed to a National Strategy on Energy Efficiency. This is a ten-year plan to accelerate the implementation of a nationwide adoption of energy-efficient practices.
In August 2017, the Government of Canada released Build Smart - Canada's Buildings Strategy, as a key driver of the Pan-Canadian Framework on Clean Growth and Climate Change.
Energy efficiency has proved to be a cost-effective strategy for building economies without necessarily increasing energy consumption. For example, the state of California began implementing energy-efficiency measures in the mid-1970s, and during the following years, California's energy consumption remained approximately flat on a per capita basis while national US consumption doubled.
Biological thermodynamics is a science that explains the nature and general laws of thermodynamic processes occurring in living organisms as nonequilibrium thermodynamic systems that convert the energy of the Sun and food into other types of energy.
Living organisms are far from equilibrium with their surrounding environmental systems, which tend towards increasing disorder (increasing entropy). Organisms must free themselves from high entropy to maintain their cellular structures for a period of time sufficient enough to allow reproduction and the resultant offspring to reach reproductive ages.
Living systems require constant energy input to maintain their highly ordered state. Cells, for example, are highly ordered and have low entropy. In the process of maintaining this order, some energy is lost to the surroundings or transformed. So while cells are ordered, the processes performed to maintain that order result in an increase in entropy in the cell's/organism's surroundings. The transfer of energy causes entropy in the universe to increase.
Energy transformation powers change in the universe. In physical systems, maximal power may occur only at submaximal efficiency, or conversely, maximal efficiency may occur only at submaximal power. A review of power and efficiency in living systems at various levels of biological organization reveals that trade-offs (negative correlations) between power and efficiency, as expected in physical systems, chiefly occur for resource-supply systems.
Synergy (positive correlations) between power and efficiency chiefly occurs for resource use systems, which may result from increasing energy allocation to production versus maintenance as production rate increases.
The energy efficiency of living systems can be improved through various methods, such as insulating buildings, using energy-efficient appliances, and adopting energy-saving behaviours.
Urban vs Township: Understanding Legal Boundaries
You may want to see also
Frequently asked questions
Living systems obtain energy from their surroundings, consuming energy-storing molecules and converting them into usable energy to do work.
The First Law of Thermodynamics states that energy can neither be created nor destroyed, only transferred or transformed. This means that energy exists in many different forms and can be converted from one form to another, such as when plants convert sunlight into chemical energy.
The Second Law of Thermodynamics states that every energy transfer involves some loss of energy in an unusable form, resulting in a more disordered system. Living systems are highly ordered and require constant energy input to maintain their state of low entropy. As they take in energy-storing molecules and transform them through chemical reactions, they lose some usable energy in the process, increasing the entropy of their surroundings.