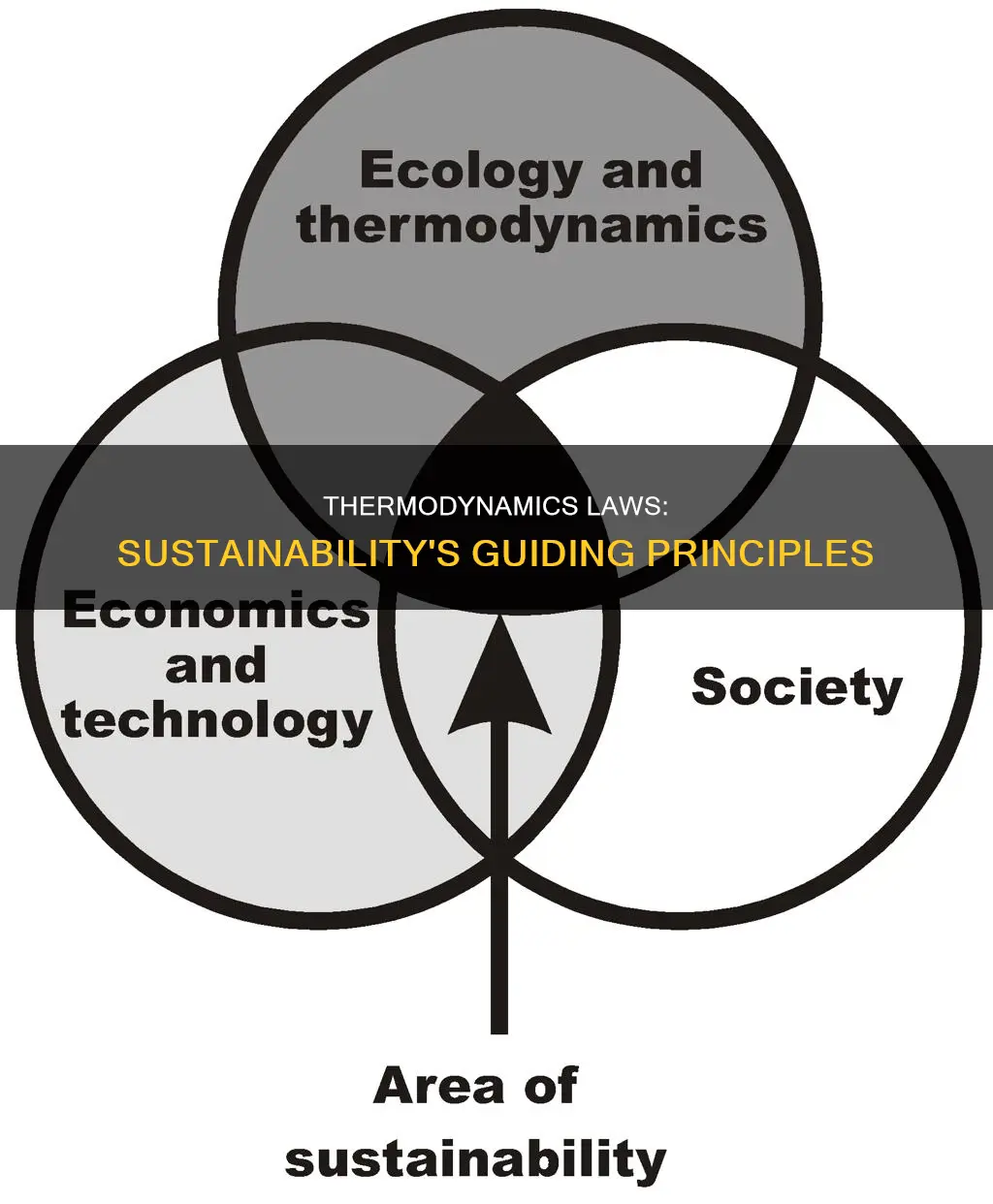
The laws of thermodynamics are foundational to our understanding of the universe and, according to Einstein, will never be overthrown. The three laws are often framed in arcane terms, but they are essential to our understanding of energy transition and sustainability. The first law states that energy cannot be created or destroyed, only transformed from one form into another. This is also known as the law of conservation of energy. The second law states that entropy, or disorder, invariably increases in a closed system. This means that an energy-consuming process will be less than 100% efficient, and the universe will progress from a state of concentrated and useful energy towards diffuse and useless energy. The third law states that a system's entropy approaches zero as its temperature approaches absolute zero, meaning it is not possible to lower the temperature of an object to absolute zero.
These laws have important implications for sustainability. For example, the concept of sustainability can be viewed through a thermodynamic lens, where thresholds can be calculated above or below which a system is either consuming too much or too little, and is therefore not in a self-preserving state of equilibrium. This approach can be used to determine whether a system can exist in a sustainable state and which factors most affect its sustainability.
The second law of thermodynamics is particularly relevant to sustainability. Renewable energy sources like solar panels and wind turbines capture diffuse and useless wind or solar energy and convert it into concentrated, useful electrical energy. While this seems to contradict the second law, it does not violate it when considering the system as a whole, which includes the sun or wind as the source of the energy. This highlights the importance of efficient energy use, as more conversion steps will amplify efficiency losses.
The laws of thermodynamics also apply to the clean energy transition. For example, fossil fuels result in the destruction of up to one-third of their own useful energy through heat transfer, while solar and wind power do not use heat transfer to deliver useful secondary energy in the form of electricity. This means that, in a clean energy system, our total primary energy consumption would significantly decrease.
Characteristics | Values |
---|---|
First Law of Thermodynamics | Energy cannot be created or destroyed |
Second Law of Thermodynamics | For a spontaneous process, the entropy of the universe increases |
Third Law of Thermodynamics | A perfect crystal at zero Kelvin has zero entropy |
What You'll Learn
The first law of thermodynamics and sustainability
The first law of thermodynamics is the law of conservation of energy. It states that energy cannot be created or destroyed. It can only be transformed from one form into another. This is an extension of the law of conservation of energy, which states that there will always be the same amount of energy in the universe as there was at the beginning.
The first law of thermodynamics is useful for dismissing myths about perpetual motion machines or the possibility of powering the world off of biomass. For example, the energy in the food we eat cannot be converted into more than the same amount of energy for our bodies to use. This is why it is not possible to generate enough energy by riding an exercise bike to power your home.
The first law of thermodynamics is also important for understanding the energy content of combustion fuels. For example, natural gas releases 304kWh of chemical energy, which can be converted into 274kWh of useful heat energy in a 90% efficient boiler.
Nuclear energy is a debatable example of the first law of thermodynamics. Nuclear energy appears to create energy from the controlled decay of Uranium-235. However, the first law of thermodynamics is upheld by claiming that all matter is really just condensed energy (via the law of special relativity, E = mc^2).
Natural gas flaring is another debatable example. Here, the useful energy in the gas appears to be destroyed as it is wasted. However, the first law of thermodynamics states that the energy is not destroyed but rather converted into heat, which then leaks into the atmosphere and eventually escapes into outer space.
The first law of thermodynamics is also relevant to sustainability. Consumption of resources by a society or other complex system can be viewed as a flow of exergy, or the useful "work" a system can do. When approached in this way, the concept of sustainability becomes a question of thermodynamics where thresholds can be calculated above or below which a system is either consuming "too much" or "too little" and is therefore not in a self-preserving state of equilibrium.
Enrico Sciubba of the Department of Mechanical and Aerospace Engineering, at the University of Roma La Sapienza, and physicist Federico Zullo of the University of Roma Tre, in Italy, have developed a simplified thermodynamic model of sustainability. Their model is an approximation of how complex systems, whether economic or ecological, behave under different conditions. It provides the foundations for building a more sophisticated representation that can be used to determine whether a system can exist in a sustainable state.
Castle Law and Motorcycles: What's the Verdict?
You may want to see also
The second law of thermodynamics and sustainability
The second law of thermodynamics states that entropy increases in a closed system. Entropy is a measure of disorder, randomness, or uncertainty. In other words, the second law of thermodynamics tells us that the universe is always moving towards a state of greater disorder and randomness. This has important implications for sustainability.
The second law of thermodynamics can be applied to sustainability through the concept of exergy, which is the useful "work" a system can do. By viewing a society or complex system as a flow of exergy, we can calculate thresholds above or below which a system is consuming "too much" or "too little," respectively, and is therefore not in a self-preserving state of equilibrium. This framework allows us to quantify sustainability and determine whether a system can exist in a sustainable state.
Enrico Sciubba and Federico Zullo have developed a simplified thermodynamic model of sustainability based on this concept. Their model considers a single population in a system using non-renewable resources, energy, and materials. By calculating the exergy rate, they can build a set of thermodynamic equations that model the population's birth and death rates and resource use. This model provides a foundation for understanding how complex systems behave under different conditions and can inform policy decisions by identifying the factors that impact sustainability the most.
The second law of thermodynamics also highlights the importance of efficient energy use. Each additional conversion step and process will result in efficiency losses, amplifying the overall efficiency losses. Therefore, to minimize waste and maximize the useful work obtained from energy sources, it is crucial to reduce the number of unnecessary conversion steps.
Furthermore, the second law of thermodynamics aligns with the principle of the Conservation of Change, which is derived from the basic principles of thermodynamics. This principle suggests that to live sustainably, we must emulate the cycles observed in nature and adapt to natural variability. Human activities that resist change and aim for highly structured and uniform outcomes, such as monocultures in agriculture, can lead to degeneration and degradation by disrupting the regenerative capacity of living systems. On the other hand, activities that embrace diversity and change, such as shifting cultivation and crop rotation, have higher regenerative potential and are more aligned with the second law of thermodynamics.
Exploring Safe Haven Laws: Fathers' Rights and Responsibilities
You may want to see also
The third law of thermodynamics and sustainability
The third law of thermodynamics states that a system's entropy approaches zero as its temperature approaches absolute zero (-273°C). This means that it is impossible to cool an object to absolute zero. According to the second law of thermodynamics, more entropy will be created outside of the cooled substance than is removed from it, meaning cooling can never be 100% efficient.
The third law is more removed from everyday energy use, but it is still relevant to certain processes. For example, liquefying natural gas to -160°C emits 15-25kg of CO2 per boe of energy in the gas, which is equivalent to around 5-7% of the energy in the natural gas. Similarly, liquefying hydrogen at -253°C and transporting it would absorb around half of the energy content in the hydrogen.
The third law of thermodynamics was developed by chemist Walther Nernst between 1906 and 1912 and is, therefore, often referred to as the Nernst heat theorem. It changed the law from a fundamental one (justified by experiments) to a derived law (derived from even more basic laws) with the development of statistical mechanics.
The third law provides an absolute reference point for determining the entropy of a system at any temperature. The entropy of a closed system, determined relative to this zero point, is the absolute entropy of that system. Mathematically, the absolute entropy of any system at zero temperature is the natural log of the number of ground states times the Boltzmann constant kB = 1.38×10^-23 JK^-1.
The third law is equivalent to the statement that it is impossible to reduce the temperature of any closed system to absolute zero in a finite number of operations. This is because, at absolute zero, a system is in a state with the minimum possible energy, and cooling a system to this state would require an infinite number of steps or an infinite amount of time.
The third law also has implications for sustainability. For example, in the context of energy and resource use, the concept of sustainability can be viewed through the lens of thermodynamics, where thresholds can be calculated above or below which a system is either consuming "too much" or "too little" and is therefore not in a self-preserving state of equilibrium.
The Reasonable Person Standard: How Does It Affect Colorado Law?
You may want to see also
Entropy and sustainability
The laws of thermodynamics offer a framework for understanding sustainability, with the second law, in particular, being highly relevant. This is the law of entropy, which states that in a closed system, entropy or disorder, invariably increases. Entropy is a measure of the ratio of heat energy flux to absolute temperature.
Enrico Sciubba and Federico Zullo have developed a thermodynamic model of sustainability that views a society's consumption of resources as a flow of exergy, or the useful work a system can do. This model allows for the calculation of thresholds above or below which a system is either consuming too much or too little and is therefore not in a self-preserving state of equilibrium. While this model does not consider social, ethical, political, or medical factors, it provides a mathematical basis for understanding sustainability and determining which aspects of a system have the most significant impact on it.
The second law of thermodynamics, therefore, encourages efficient energy use with as few unnecessary conversion steps as possible. Each additional conversion step will result in efficiency losses, amplifying the overall amount of energy wasted. This is particularly relevant for green hydrogen, which is predicted to waste around 50% of the renewable energy used in its production due to various energy penalties.
In summary, the law of entropy highlights the importance of embracing change and diversity in natural systems to maintain sustainability. It also provides a mathematical framework for understanding sustainability and encourages efficient energy use to minimise entropy losses.
Labor Laws for 17-Year-Olds in Missouri: What You Need Know
You may want to see also
Exergy and sustainability
Exergy is a thermodynamic quantity that can be used to assess and improve energy systems. It is defined as the maximum amount of work that can be produced by a system or a flow of matter or energy as it comes to equilibrium with a reference environment.
Exergy analysis can be used to better understand the benefits of green energy technologies and identify efficiency improvements and reductions in thermodynamic losses. It provides more useful and meaningful information than energy analysis, which can be misleading and confusing when used to assess the benefits of green energy and technologies. For example, in a geothermal power plant using geothermal liquid water at 160 °C and producing 15 MW of net power, energy analysis shows that the energy efficiency of the plant is 6%. However, exergy analysis reveals that the plant's exergy efficiency is actually 34%, with 66% of the input exergy being lost.
The use of exergy can help to increase the utilisation of more environmentally benign and sustainable energy and technologies by making their benefits clear to experts and non-experts alike. This includes engineers, scientists, decision-makers, the public, the media, and industry and government officials. By providing a clearer understanding of efficiency improvements and reductions in thermodynamic losses, exergy can play a crucial role in the transition to green energy and technologies.
A simplified thermodynamic model of sustainability has been developed by Enrico Sciubba and Federico Zullo, which approaches the consumption of resources by a society or complex system as a flow of exergy. This model provides a mathematical basis for determining which aspects of a system affect its sustainability and can help policymakers focus on the most important factors. It also allows for the calculation of thresholds above or below which a system is either consuming too much or too little and is therefore not in a self-preserving state of equilibrium.
Security Personnel: Understanding Arrest Laws and Their Application
You may want to see also
Frequently asked questions
The first law of thermodynamics states that energy cannot be created or destroyed. It can only be transferred or transformed from one form to another.
The first law of thermodynamics can be applied to sustainability by viewing the consumption of resources by a society or complex system as a flow of exergy, or the useful "work" a system can do. This allows for the calculation of thresholds above or below which a system is either consuming too much or too little and is therefore not in a self-preserving state of equilibrium.
The second law of thermodynamics states that entropy, or the amount of disorder in a closed system, will always increase over time.
The second law of thermodynamics can be applied to sustainability by encouraging efficient energy use, as more conversion steps and processes will amplify efficiency losses.
The third law of thermodynamics states that as the temperature of a system approaches absolute zero (-273°C), the system's entropy will approach zero, meaning that it is not possible to lower the temperature of an object to absolute zero.