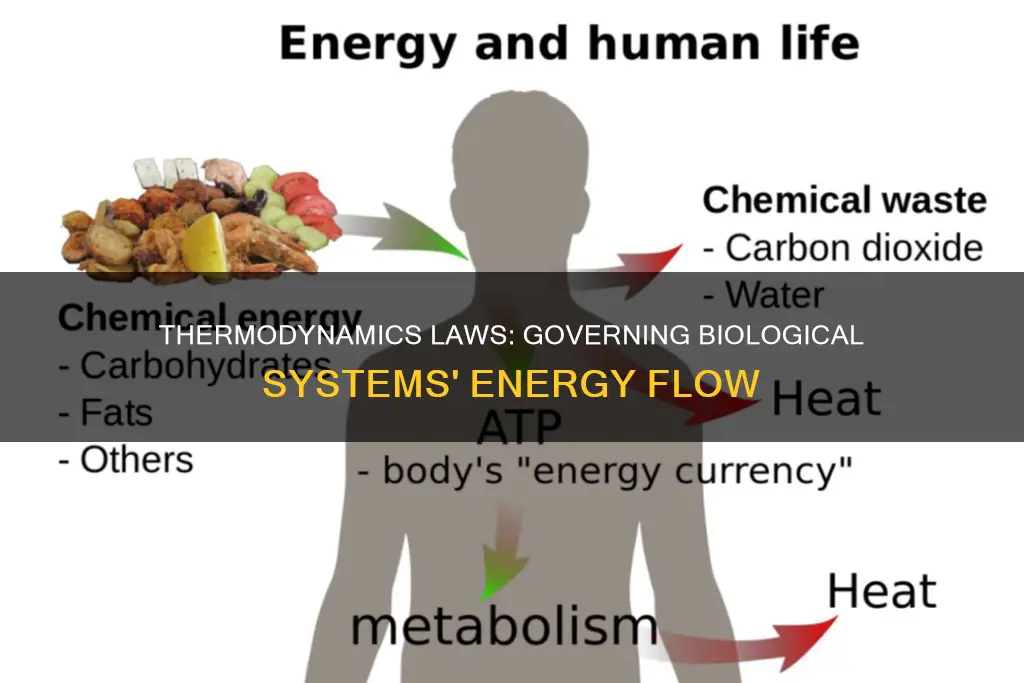
The laws of thermodynamics are a set of principles that govern the transfer of energy in all systems in the universe. They are important unifying principles of biology, governing the chemical processes (metabolism) in all biological organisms. The First Law of Thermodynamics, also known as the law of conservation of energy, states that energy can neither be created nor destroyed. In a closed system, the energy remains constant but may change from one form to another. The Second Law of Thermodynamics states that when energy is transferred, there will be less energy available at the end of the transfer process than at the beginning. This is due to entropy, which is a measure of disorder in a closed system. As energy is transferred, entropy increases.
Biological organisms are open systems, meaning they can exchange energy with their surroundings. They require a constant input of energy to maintain their highly ordered state. The laws of thermodynamics are relevant to biological systems because they govern the transfer and transformation of energy in these systems. For example, plants perform one of the most biologically useful energy transformations on Earth: converting the energy of sunlight into the chemical energy stored within organic molecules through photosynthesis. Animals, on the other hand, cannot generate energy directly from sunlight and must consume plants or other animals for energy.
The application of the laws of thermodynamics to biological systems has led to the development of fields such as biological thermodynamics, which explains the nature and general laws of thermodynamic processes occurring in living organisms. It also includes the study of concepts such as entropy and its relationship to evolution, genetic instructions, and disease states. Overall, the laws of thermodynamics provide a fundamental framework for understanding the energy transformations and mass distributions that occur in biological systems.
What You'll Learn
- The First Law of Thermodynamics states that energy can be transferred or transformed but cannot be created or destroyed
- The Second Law of Thermodynamics states that entropy increases in a closed system
- Living organisms are open systems, exchanging energy with their surroundings
- The Gibbs equation describes the relationship between the change in free energy, enthalpy, temperature, and entropy
- Activation energy is required to overcome the energy requirement barrier to a spontaneous reaction
The First Law of Thermodynamics states that energy can be transferred or transformed but cannot be created or destroyed
The First Law of Thermodynamics, also known as the law of conservation of energy, states that energy can neither be created nor destroyed. It may change from one form to another, but the energy in a closed system remains constant. This means that energy can be transferred or transformed but cannot be created or destroyed.
The First Law of Thermodynamics deals with the total amount of energy in the universe, which is constant. In other words, there has always been, and always will be, the exact same amount of energy in the universe. Energy exists in many different forms. According to the First Law of Thermodynamics, energy can be transferred from place to place or transformed into different forms.
The First Law of Thermodynamics is an important unifying principle of biology. These principles govern the chemical processes (metabolism) in all biological organisms. All biological organisms require energy to survive. In a closed system, such as the universe, this energy is not consumed but transformed from one form to another.
Cells, for example, perform a number of important processes that require energy. In photosynthesis, the energy is supplied by the sun. Light energy is absorbed by cells in plant leaves and converted to chemical energy. The chemical energy is stored in the form of glucose, which is used to form complex carbohydrates necessary to build plant mass.
The energy stored in glucose can also be released through cellular respiration. This process allows plant and animal organisms to access the energy stored in carbohydrates, lipids, and other macromolecules through the production of ATP. This energy is needed to perform cell functions such as DNA replication, mitosis, meiosis, cell movement, endocytosis, exocytosis, and apoptosis.
The First Law of Thermodynamics is also relevant to the understanding of biological systems at the molecular level. At this level, the biosphere is an immense biochemical gene factory containing enormous amounts of genetic instructions operating under the laws of thermodynamics. For example, functional genetic instructions (FGI) in genomes are the instructions for each respective life form. These instructions control gene expression, thus maintaining the low entropy, homeostatic state necessary for organisms to survive and reproduce.
In summary, the First Law of Thermodynamics states that energy can be transferred or transformed but cannot be created or destroyed. This law is fundamental to our understanding of biological systems, as it governs the transfer and transformation of energy in all biological organisms.
Mens Rea in Contract Law: A Relevant Consideration?
You may want to see also
The Second Law of Thermodynamics states that entropy increases in a closed system
The laws of thermodynamics are important unifying principles of biology, governing the chemical processes (metabolism) in all biological organisms. The Second Law of Thermodynamics states that when energy is transferred, there will always be less energy available at the end of the transfer process than at the beginning. This is due to the concept of entropy, which is a measure of disorder in a closed system. In a closed system, entropy increases as energy is transferred, and all of the available energy will not be useful to the organism.
Biological organisms are open systems, meaning they can exchange energy with their surroundings. They consume energy-storing molecules and release energy to the environment by doing work. However, the transfer of energy is never completely efficient, and some energy is always lost in the form of heat. This loss of energy results in an increase in disorder, or entropy, in the system's surroundings.
Living systems require a constant input of energy to maintain their highly ordered state. Cells, for example, are highly ordered and have low entropy. To maintain this order, energy is lost to the surroundings or transformed, resulting in an increase in entropy outside the cell or organism. While individual cells or organisms may have low entropy, the processes performed to maintain that order contribute to an overall increase in entropy in the universe.
The Second Law of Thermodynamics, therefore, has significant implications for biological systems. It explains why living organisms need to constantly obtain energy from their surroundings and why energy transfers within biological systems are never completely efficient. It also highlights the role of entropy in the increase in disorder or randomness within a system, which is a fundamental concept in understanding the behaviour of biological organisms.
The Earth and the Second Law of Thermodynamics
You may want to see also
Living organisms are open systems, exchanging energy with their surroundings
Biological organisms are open systems, meaning they can exchange energy with their surroundings. This is in contrast to closed systems, which cannot transfer energy to their surroundings. The stove, pot, and water used to heat water on a stove, for example, constitute an open system because heat can escape into the air.
Living organisms require a constant input of energy to maintain their highly ordered, low-entropy state. This is governed by the laws of thermodynamics, which dictate that energy can be transferred or transformed but not created or destroyed. In biological systems, energy is derived from the sun and food and converted into other types of energy.
The first law of thermodynamics, also known as the law of conservation of energy, states that energy can neither be created nor destroyed. It can change form, but the total amount of energy in the universe remains constant. This law applies to all systems in the universe, including biological organisms.
The second law of thermodynamics states that when energy is transferred, there will be less energy available at the end of the process than at the beginning. This is due to entropy, which is a measure of disorder in a closed system. As energy is transferred, entropy increases, and not all the available energy will be useful to the organism.
Living organisms maintain their ordered, low-entropy state by exchanging energy with their surroundings. They consume energy-storing molecules and release energy through work, such as cellular functions like DNA replication and cell movement. This exchange of energy with the surroundings is essential for the survival of living organisms.
The challenge for living organisms is to obtain energy from their surroundings in forms that they can transform into usable energy to perform work. This involves converting energy from one form to another, such as converting the energy of sunlight into chemical energy stored within organic molecules. This process is not completely efficient, and some energy is lost as heat or other unusable forms.
The continuous input of energy is necessary to counteract the natural tendency towards disorder, as dictated by the second law of thermodynamics. By exchanging energy with their surroundings, living organisms can maintain their ordered, low-entropy state and perform the functions necessary for survival.
California Laws: Are They Applicable in Sslab City?
You may want to see also
The Gibbs equation describes the relationship between the change in free energy, enthalpy, temperature, and entropy
The laws of thermodynamics govern the chemical processes, or metabolism, in all biological organisms. The First Law of Thermodynamics, also known as the law of conservation of energy, states that energy cannot be created or destroyed, only transferred or transformed. The Second Law of Thermodynamics states that when energy is transferred, there will always be less energy available at the end of the process than at the beginning, due to the presence of entropy. Entropy is a measure of disorder in a closed system, and it increases as energy is transferred.
Biological organisms are open systems, meaning they can exchange energy with their surroundings. They consume energy-storing molecules and release energy in the form of work. The challenge for all living organisms is to obtain energy from their surroundings and convert it into a form that can be used to perform work.
The Gibbs equation, developed by Josiah Willard Gibbs in the 1870s, describes the relationship between the change in free energy, enthalpy, temperature, and entropy in a system. The equation is as follows:
ΔG = ΔH - TΔS
Where:
- ΔG is the change in free energy
- ΔH is the change in enthalpy
- T is the absolute temperature
- ΔS is the change in entropy
The Gibbs free energy combines enthalpy and entropy into a single value. It can be used to calculate the maximum amount of work that can be performed by a closed system at a constant temperature and pressure. The change in free energy, ΔG, can also predict the direction of a chemical reaction under constant temperature. If ΔG is positive, the reaction is non-spontaneous, meaning external energy input is required for the reaction to occur. If ΔG is negative, the reaction is spontaneous and will occur without external energy input.
The sign of ΔG indicates the direction of a chemical reaction and whether it is spontaneous or not. If ΔG is negative, the reaction is spontaneous in the direction written. If ΔG is zero, the system is at equilibrium, and there is no net change in either the forward or reverse direction. If ΔG is positive, the reaction is not spontaneous, and external energy input is needed for the process to occur.
The Gibbs equation is a valuable tool for understanding the relationship between free energy, enthalpy, temperature, and entropy in biological systems. It helps determine the spontaneity and direction of chemical reactions, providing insights into the energy transformations that occur within living organisms.
Ohms Law and Light Bulbs: An Exception Explained
You may want to see also
Activation energy is required to overcome the energy requirement barrier to a spontaneous reaction
The laws of thermodynamics are fundamental to our understanding of biological systems. The first law of thermodynamics, also known as the law of conservation of energy, states that energy cannot be created or destroyed, only transformed from one form to another. This principle applies to biological organisms, which require a constant energy input to survive. For example, during photosynthesis, plants convert sunlight into chemical energy stored in glucose, which is then used for various cellular functions.
The second law of thermodynamics states that when energy is transferred, there will always be a loss of energy in the form of heat. This is important in biological systems because it means that energy transfers are never completely efficient. For instance, in cellular metabolic reactions, some energy is lost as heat, which helps warm-blooded animals maintain their body temperature.
Now, let's delve into the concept of activation energy and its role in spontaneous reactions within biological systems. Activation energy refers to the minimum amount of energy required for reactants to undergo a chemical reaction. It represents the energy barrier that must be overcome for the reaction to occur at a reasonable rate. The concept was introduced by Swedish scientist Svante Arrhenius in 1889.
In the context of biological systems, activation energy is crucial for various chemical reactions that take place within living organisms. For example, during cellular respiration, activation energy is needed to convert the energy stored in carbohydrates, lipids, and other macromolecules into a form that cells can use to perform essential functions such as DNA replication and cell movement.
The presence of an energy barrier does not necessarily contradict the spontaneity of a reaction. Spontaneous reactions are characterized by having a negative delta G, indicating that the final state has lower free energy than the initial state. While an energy barrier exists, the total energy released by the reaction exceeds the energy required to overcome this barrier, making the formation of products favorable.
Furthermore, the activation energy can be influenced by catalysts or enzymes, which facilitate the reaction without being consumed in the process. They achieve this by providing a more favorable environment for the reaction to occur, reducing the amount of energy required to reach the transition state. This, in turn, increases the rate of the reaction.
In summary, activation energy is a critical concept in understanding how chemical reactions occur in biological systems. It represents the energy requirement that must be met for a reaction to proceed, and it plays a role in determining the rate at which the reaction occurs. While spontaneous reactions have a negative delta G, indicating a favorable energy difference between the initial and final states, they may still encounter an energy barrier that needs to be overcome for the reaction to take place. Catalysts and enzymes can assist in this process by lowering the activation energy needed.
Employment Laws: Independent Contractors' Rights and Responsibilities
You may want to see also
Frequently asked questions
The First Law of Thermodynamics, also known as the law of conservation of energy, states that energy can neither be created nor destroyed. It may change from one form to another, but the energy in a closed system remains constant. This means that energy can be transferred or transformed, but the total amount of energy in the universe is always the same. Biological organisms are open systems that require a constant input of energy to survive. They take in energy-storing molecules and transform them through chemical reactions, losing some usable energy in the process.
The Second Law of Thermodynamics states that when energy is transferred, there will be less energy available at the end of the transfer process than at the beginning due to entropy, the measure of disorder in a closed system. Entropy increases as energy is transferred, and all energy transfers result in the loss of some usable energy. Biological systems are highly ordered and require constant energy input to maintain their state of low entropy. While living things are highly ordered, the entropy of the universe as a whole is constantly increasing due to the loss of usable energy with each energy transfer that occurs.
Living organisms are programmed by functional genetic instructions (FGI) to grow and reproduce by maintaining a variety of hemistable, ordered structures (low entropy). They are far from equilibrium with their surrounding environmental systems, which tend towards increasing disorder (increasing entropy). Organisms must free themselves from high entropy to maintain their cellular structures for a period of time sufficient for reproduction. The laws of thermodynamics govern energy transformations and mass distributions in biological systems, and functional genetic instructions control gene expression to maintain the low entropy, homeostatic state necessary for survival and reproduction.